Sponsored By
News
Splash art for the match 3 game Clockmaker.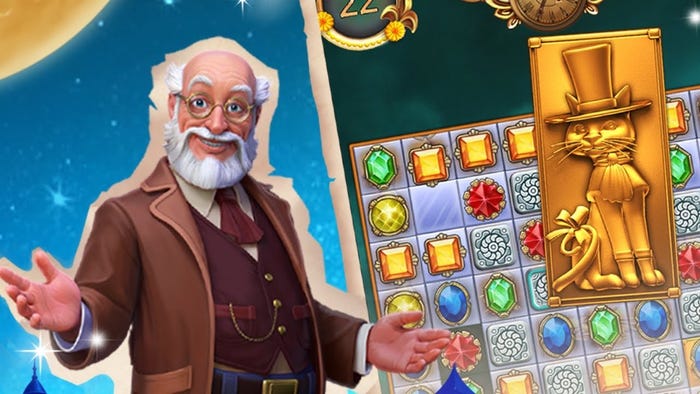
Business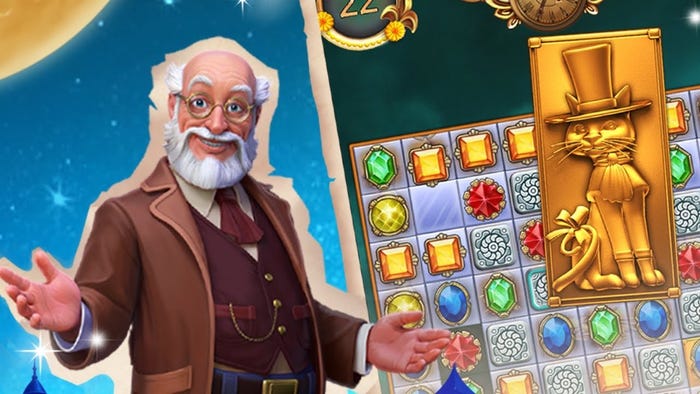
Clockmaker dev Belka Games cuts 20 percent of staffClockmaker dev Belka Games cuts 20 percent of staff
The 33 employees were let go across various teams, and as part of Belka's larger business adjustment.
Daily news, dev blogs, and stories from Game Developer straight to your inbox